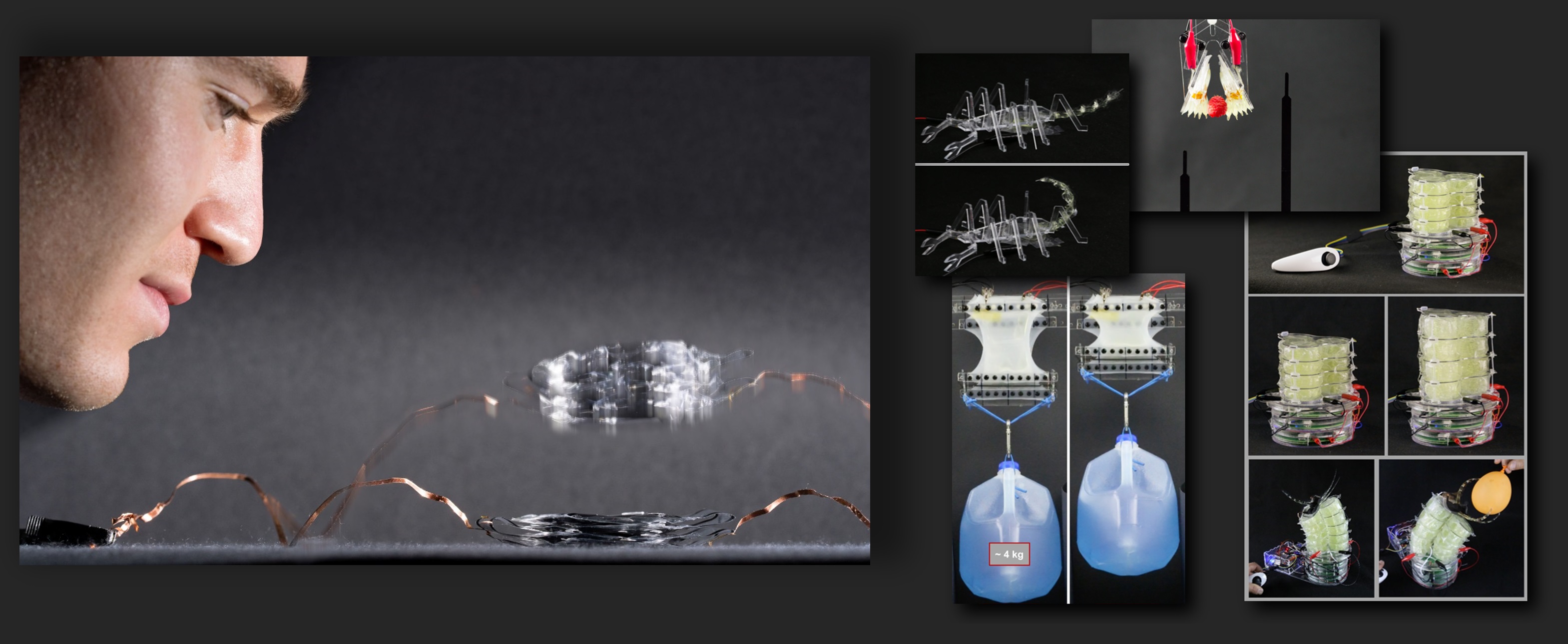
Zachary Yoder testing a high power-to-weight ratio stack of HASEL artificial muscles – a new platform technology pioneered by us.
Today’s robotic systems excel at precise and repeatable tasks, and have become widespread in manufacturing and industrial environments. However, as demand grows for versatile and multi-functional robots, and as the physical distance between humans and robots continues to diminish, the rigid links and electric motors that make up these traditional robotic systems are becoming a limiting factor. The sensors and controls required to make them safer to operate around humans are complex, expensive, and error-prone, and their non-adaptable nature reduces their effectiveness in unpredictable environments.
Yet nature provides countless examples of organisms that can easily carry out tasks considered highly challenging for today’s robots, such as manipulating a variety of objects or traversing varying terrain. These organisms are driven by soft muscles and surrounded by conformal, sensor-rich skin – a far cry from the electric motors and rigid links that make up traditional robotic systems. Biological muscle in particular is a masterpiece of evolution, as it powers the ultra-versatile arms of an octopus, is strong enough to move an elephant and fast enough for the wings of a hummingbird, self-heals after damage, and is seamlessly integrated with distributed sensing. Not surprisingly, these astonishing capabilities have inspired the creation of artificial muscles, which has been considered a grand challenge of science and engineering for centuries, dating back to the 1600’s when Robert Hooke first recorded the idea, all the way to very recent papers appearing frequently in top journals.
Consequently, one of our central goals in this main field of research is the development of new classes of actuators – key components of all robotic systems – that replicate the sweeping success of biological muscle and that transform our ability to design robotic systems for entirely new fields of application. Our current research focus is to understand the fundamental principles and mechanisms, as well as robotics applications of a new class of self-sensing, high-performance artificial muscles, termed hydraulically amplified self-healing electrostatic (HASEL) actuators, a platform technology pioneered by us. HASEL artificial muscles feature high speed and efficiency as well as the capability to self-heal from electrical damage, and they harness a new electrohydraulic mechanism, where electrostatic Maxwell stress activates soft, hydraulic structures to achieve a wide variety of actuation modes. HASEL actuators show promise to become the first technology where all performance metrics and capabilities match or exceed those of biological muscle, thus being a prime candidate to solve a centuries-old grand challenge. Further, modeling results lay out a roadmap showing how to drastically improve performance of HASEL actuators, surpassing both biological muscle and traditional electromagnetic motors. With rich underlying physics and chemistry to be further explored, the HASEL technology is poised to create a vibrant new field of research that promises a variety of practical applications that will improve human quality of life
Overall, in a broader and longer-term context, our research in this main field is driven by projects that simultaneously aim to solve practical problems in robotics and to answer the following fundamental research questions:
- What are the fundamental physical limits of electrical (or chemical/thermal/etc.) to mechanical energy conversion in robotic actuation systems? How close can specific actuation principles based on specific materials system get to those fundamental limits?
- Can we match or exceed performance of biological muscle with new actuation technologies to allow revolutionary new designs of bio-inspired intelligent systems across different length-scales?
- Which physical principles and mechanisms for transducers are versatile enough to be used in highly adaptable soft robotic systems, and which ones can mimic survival features such as self-healing and tight integration with sensing, as seen in biology?
- Can we exceed performance of traditional electromagnetic servo motors with new actuation technologies based on entirely new principles?
- Which physical principles and mechanisms for actuators allow the highest possible values for power/weight (or volume), energy/weight (or volume), energy efficiency and bandwidth of actuation?
- For specific actuation principles, can we push the underlying materials systems far beyond currently identified limits and maxima?
- What controls or machine learning methods will allow the creation of autonomous, bioinspired intelligent systems built from robotic materials, that can achieve levels of dexterity, agility and adaptability currently only seen in natural organisms?